Corrosion is the breakdown of materials due to chemical reactions. It is usually oxidation with air molecules and often in the presence of water. Corrosion also occurs when an acidic/basic corrosive substance touches another material.
Steel and concrete have become the most common materials for manmade structures over the last hundred or so years, with the use of the composite material, concrete reinforced with steel, becoming one of the most popular methods for civil construction.
The historical reasons for steel-reinforced concrete’s popularity are not hard to find: its cheapness, high structural strength, mouldability, fire resistance, and supposed imperviousness to the external environment while requiring little or no maintenance, provide a virtually unbeatable combination.
To harness these properties, both national and international standards have been developed. The standards for concrete and steel were initially defined principally by compositional limits and strength, which has continued to be the primary quality control.
Until the 1950s, it was assumed that when steel was encased in the alkaline concrete matrix, neither would suffer from any degradation for the indefinite future. However, evidence of degradation was noted as early as 1907 (Knudsen, 1907), where it was observed that chlorides added to concrete could allow sufficient corrosion of the steel to crack the concrete.
The implicit assumption by many civil engineers of reinforced concrete’s virtually infinite durability has proven to be true in several cases, with structures reaching their design lives without any evidence of structural degradation. However, it is now evident that in areas with an aggressive atmosphere, the concrete can be damaged, or the steel can corrode dramatically shorter than the specified design life.
Also Read: What is Civil Engineering? | History and Functions
The current design life was initially set at 120 years for UK highways. Despite all the evidence of highway structures showing significant problems after a short period, it is still set at this extremely hopeful figure even though no corrosion design life analysis is required.
This head-in-the-sand approach can be contrasted with the reality illustrated by research (Bamforth, 1994) showing that the estimated time to corrosion activation of steel reinforcement in modern concrete with the designated cover can be as low as five and a half years at the 0.4% chloride level with modern concrete.
These research findings are in good accordance with site investigations. A substantial number of structures have been found to have their steel reinforcement sufficiently corroded within 20 years of construction to be structurally unsound.
Even after the publicity surrounding the large number of structures exhibiting acute signs of distress 25 or so years into a designed 120-year life-span, there is still a body of engineers who believe that all that is required to achieve any specified design life in a hostile environment is to provide a higher concrete grade with the same design and maintenance of the structure.
This contention does not correspond with the facts and means that publications such as this book will deal with civil engineering miscalculations of the past and those perpetrated in the future.
The traditional use of cathodic protection has been to prevent corrosion of steel objects in the ground or water, which is still its most common application. It is almost universally adopted on ships, oil rigs, and oil and gas pipelines. Over the last 50 years, cathodic protection has advanced from being a black art to something approaching a science for these applications.
Over the past 30 or so years, there has been a steady increase in the use of cathodic protection for rehabilitating reinforced concrete structures exhibiting signs of distress.
The most common damage mechanism is chloride-induced corrosion of the steel reinforcement; this is usually what cathodic protection systems are intended to stop.
Initially, the cathodic protection techniques for reinforced concrete followed the practice of ‘traditional’ impressed current systems closely but, particularly over the past decade or so, there have been significant developments that have allowed the protection of concrete structures to become a legitimate and yet distinctly different part of the cathodic protection mainstream with its protection criteria, anode types, and even power supplies.
The object of this volume is to introduce the current state of the art in the cathodic protection of concrete and outline other related electrochemical techniques for stopping the corrosion of steel reinforcement.
Some guidelines on what cathodic protection is and how and when to use it are also discussed so that a practicing civil engineer or owner should have an introduction into the murky world of the cathodic protection of reinforced concrete.
Also Read: Best Types of Kitchen | All You Need To Know- Right Now
Electrochemical Corrosion
Electrochemical reactions are widely used by mankind for industrial processes such as anodizing or chloride production. They are used directly by most people every day of their lives when using a battery.
A surprising number of engineers vaguely remember an explanation in chemistry classes of how a battery operates. This is normally reiterated as being about electrolytes with ions swimming about, with anodes and cathodes making an appearance, and then dismissed as not important in ‘proper’ civil or mechanical engineering.
Unfortunately for those who do not like electrical circuits, corrosion is also an electrochemical process and is of great economic importance, as those with old cars will testify, and has been estimated to consume 4% of the Gross National Product of, for example, the United States (Bennett et al., 1978). This percentage is likely to be of the same order globally.
In all low-temperature corrosion reactions and the processes given above, an electrochemical cell is needed for the reactions to occur. This cell comprises an anode and cathode separated by an electrolytic conductor (electrolyte) with a metallic connection.
This is shown schematically below. A practical definition of an anode is the area where corrosion occurs, while the cathode is where no corrosion occurs.
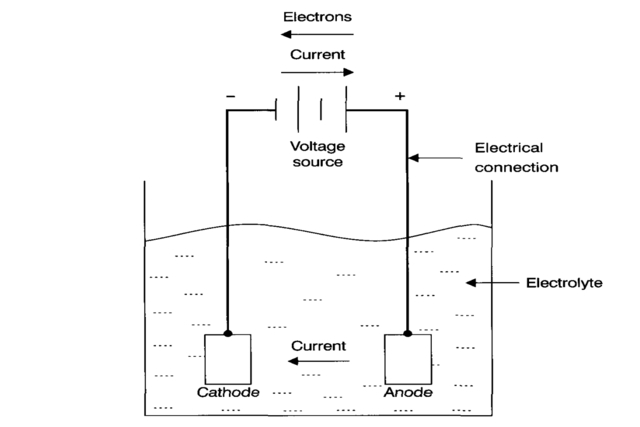
When a metal such as steel is in an electrolyte (an aqueous solution that can carry ions such as water with some rock salt in the solution), a corrosion cell can be formed. Part of the steel in the electrolyte forms the anode, and part in the same electrolyte forms the cathode. Corrosion, in this case, would occur at all the anode points dispersed around the steel (see Figure below). This gives the appearance of general or uniform corrosion.
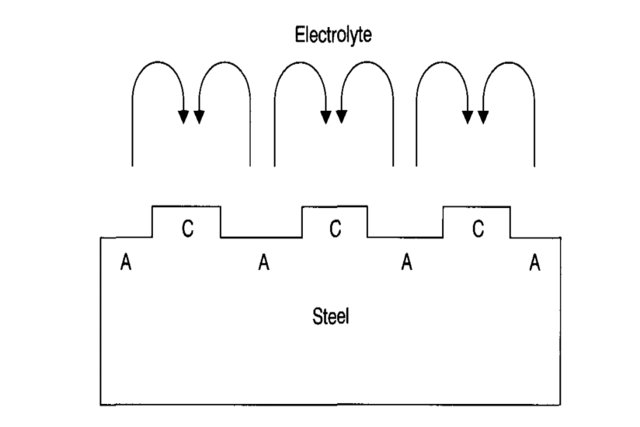
If steel is physically attached (i.e., welded, bolted, or cast) to a piece of zinc and both are placed in an electrolyte, then the zinc will form all the anode, and the steel will only form the cathode.
The result of this will be that all the corrosion reactions will occur to the zinc, which will be consumed, and a balancing reduction reaction (non-corrosion) will occur to the steel, which will not be affected by its immersion in the electrolyte.
This is the basis of cathodic protection. When you cathodically protect steel or any other metal, you change it from acting either as an anode or both an anode and cathode to acting totally as a cathode. This is done by the imposition of an external anode which will corrode preferentially (see Figure below).
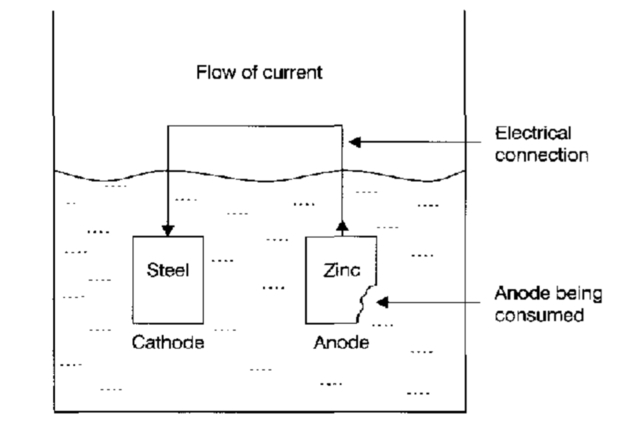
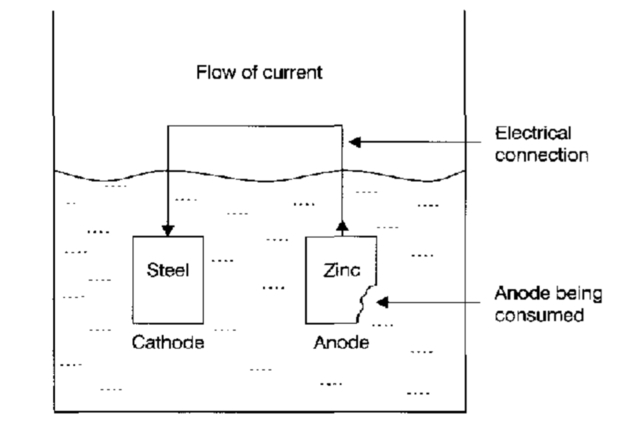
Also Read: Engineer | Definition, and History You Should Know Right Now
Corrosion of Steel
In common with all engineering metals, steel is intrinsically unstable in that it wants to return to its stable state where it came from as an ore.
The result of this reversion is rust (commonly iron oxide, but it can be iron sulfide or other compounds) which, while having considerably greater chemical stability, also has considerably reduced mechanical properties, such as strength, compared with the original steel.
With this tendency to corrode, the principal question is not whether steel will rust but how fast it will rust.
The environment and the stability of the oxide layer on the surface usually decide the corrosion rate of steel. The reaction rate is slow if this layer forms a protective skin that is not breached.
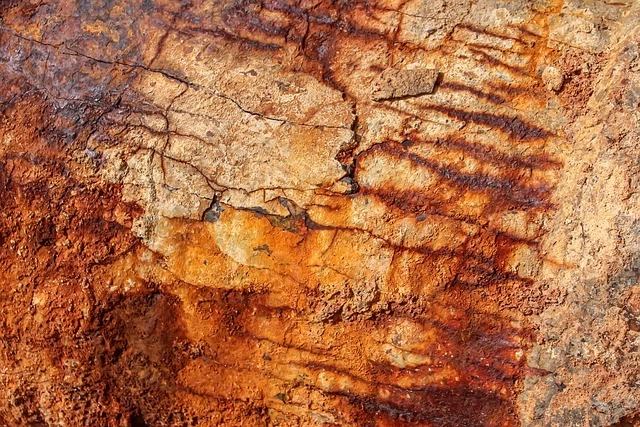
If, however, the oxide layer is opened at many places and sloughs off the surface, providing access for more oxygen (which is usually dissolved in water) to the unreacted steel surface. A high corrosion rate can be expected.
Straight carbon and high-yield steels are the most commonly used grades for rebar in normal civil engineering projects. Neither of these types has a particularly protective oxide film, and both rely on the alkalinity of the concrete to stabilize this skin.
There will be a rapid color change when steel corrodes in a standard atmosphere, i.e., outdoors. This is known as ‘flash’ resting. For example, blast-cleaned steel in a moist environment changes color when the contractor finishes the blasting operation and opens the paint pots.
This rusting is evidenced by a change in the surface color from silver to orangey red over all the exposed surfaces. In this case, the corrosion is very rapid because of the presence of ample fuel (oxygen) and the absence of a protective oxide film.
In a saline environment, the flash rusting is even quicker as chloride helps the water to conduct current. If the steel were examined visually under a microscope, it would all look the same color, as in this case, the individual anode and cathode sites are very small, perhaps within a few microns of each other.
In cases where the steel is exposed directly to the atmosphere and is at a normal (neutral) pH, and there is a reasonable supply of oxygen, there will be widespread and uniform corrosion.
This is normally observed when large steel sections rust and can be seen on any uncovered steel article, particularly on beaches and other places with an aggressive atmosphere. An example is given in the Figure above.
When oxygen access to the steel is reduced, which becomes the corrosion limiting step, i.e., when sufficient aggressive ions are present at the steel interface so that the corrosion reaction can happen very quickly, other forms of corrosion may occur.
The most common is pitting corrosion. This, for example, will occur when a surface coating on the steel is breached, allowing oxygen and moisture access to a relatively small area. In older cars, these are commonly seen as rust spots. This situation is shown schematically in the Figure below.
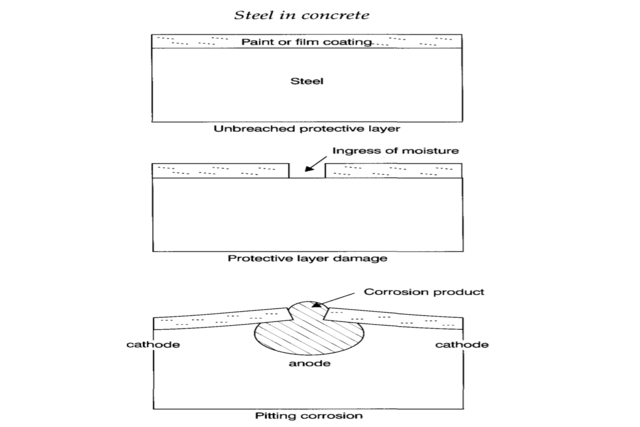
Steel in Concrete
Concrete typically provides embedded steel with a high degree of protection against corrosion. One reason for this is that cement, which is a constituent of concrete, is strongly alkaline.
This means that the concrete surrounding the steel provides an alkaline environment for the steel. This stabilizes the oxide or hydroxide film and thus reduces the oxidation rate (corrosion rate) of the steel.
This state with a very low corrosion rate is termed passivation. The other reason that concrete provides embedded steel with protection is that it provides a barrier to the outside elements, which are aggressive to the steel.
The chloride ion is the most common agent for the depassivation of steel in concrete.
The traditional wisdom was that concrete of a low water-cement ratio that was well cured would have a sufficiently low permeability to prevent significant penetration of corrosion-inducing factors such as oxygen, chloride ions, carbon dioxide, and water. Unfortunately, this is not the proper position.
Some of this can be explained by the fact that concrete is inherently porous, whatever its composition, and if there is a concentration gradient, then at some time, a sufficient quantity of aggressive ions will be passed through the concrete to initiate corrosion.
The crux is at ‘some time’ as this might be sufficiently long to achieve the design life, or it might not. Another reason is probably that cracks exist on all full-scale structures, providing preferential pathways for corrosion-inducing factors.
Fortunately, in most steel-reinforced concrete structures, corrosion does not occur in the design life. However, this is a situation that occurs more by luck than judgment.
With concrete of a suitable quality, corrosion of steel can be prevented for a certain period, provided that the structure or element is properly designed for the intended environmental exposure.
In severe exposure, such as in bridge decks exposed to deicing salts or pilings in seawater, the permeability of concrete conforming to certain standards has been measured, and the time required for the onset of corrosion using this data has been calculated as shown in the Table below.
Concrete | Minimum cover (mm) | With a chloride concentration of 0.4% | With a chloride concentration of 1% |
Old pre-cast standard | 38 | 23.7 | 53.8 |
BS 8110 (general) | 50 | 5.5 | 12.4 |
BS 5400 (bridges) | 65 | 9.2 | 20.9 |
BS 6349 (maritime) | 75 | 12.3 | 27.9 |
As can be seen, a corrosion initiation period of only five and a half years is possible when using modern concrete in compliance with the current standard in a particular environment.
Where the concrete quality and cover of a new structure are insufficient to provide the required design life, several measures may be used, such as corrosion inhibitors, coatings on the steel, coatings on the concrete, or cathodic protection.
The correct choice should be determined after evaluating all the options for their cheapness, reliability, and effectiveness over the design life of their structure.
If the structure is not properly corrosion-designed for the anticipated environment, or if the environment and other factors were not as anticipated or changed during the structure’s life, there might be problems.
Instances of distress due to corrosion can be found in almost all applications of modern reinforced or prestressed concrete; buildings, silos, beams, bridge decks, piles, other supports, tanks, and pipes are some common examples.
Normally the first evidence of distress is brown staining of the concrete surface nearest to the corroding embedded steel. This staining is caused by the permeation of iron ions through microcracks in the concrete to the surface and is often accompanied by the macrocracking of the concrete shortly afterward.
The cracking occurs because certain steel corrosion products, such as iron oxides, have a substantially greater volume than the metallic element (iron) from which it was formed. The forces generated by this expansive process exceed the tensile strength of the concrete with resulting cracking.
Steel corrosion not only causes structural distress or disfigurement because of staining, cracking, and spalling of the concrete. It also reduces the compressive strength of the structure (because of the damaged concrete) but often, more critically, can cause structural failure due to the reduced cross-section and hence reduced tensile capacity of the steel.
This reduced tensile capacity usually is only significant in localized areas and is more structurally critical with prestressing steel tendons than reinforcing bars. This is because the load cannot be easily redistributed in a pre-or post-tensioned structure, unlike cast in-situ structures.
As an example of the extreme damage which can occur with steel in cracked and stained concrete, it is common to find that 60 or 70% of the cross-section has corroded through. All concrete corrosion engineers have their apocryphal stories describing structures where there has been a 100% section loss of the steel reinforcement. In certain circumstances, this can be seen at many points.
Mechanisms
When reinforcement steel in concrete corrodes, the process is similar to taking power from an ordinary battery. When steel corrodes in a battery, a metal dissolves, producing a small current between the +pole and the • pole.
For steel reinforcement corroding in the concrete, one tiny area is the +pole (anode), and another much more extensive area is the pole (cathode).
The corrosion current flows out of the steel at the anode, the part corroding, through the concrete and into another part of the steel where there is no corrosion occurring, i.e., the cathode. This flow is called a corrosion circuit. Steel is dissolved at the anode and eventually forms iron oxide at this location.
The electrical connection between + and • can be disconnected for a battery. The circuit is then broken with the result that the current is stopped, and thus the dissolution of metal stops.
For steel reinforcement in concrete, the ionic flow running through the concrete and attachment between the steel cannot be disconnected as the corrosion circuit is buried in the structure.
Instead, it is possible by using an ‘artificial’ anode to add a new and higher current to the original corrosion circuit, which runs in the opposite direction of the corrosion current.
This makes all the previous +poles (anodes) into current receivers. Thus all the steel reinforcement is made into a negative pole, i.e., cathodic, hence the name ‘cathodic protection.’
In electrochemical corrosion, a flow of electrical current and one or more chemical processes are required for metal loss. The flow of electrical current can be caused by ‘stray’ electrical sources such as from a train traction system or from significant differences in potential between parts of the structure caused by factors such as differential aeration from the movement of sea-water (the mechanism for this is still uncertain, but it could be that very large cathode area are built up in the tidal zone
because of oxygen charging).
Electrochemical corrosion by these electrical current sources is rare but can be serious when it occurs. Often this process can contribute to corrosion when other aggressive factors occur.
The passivation on the steel by the alkalinity would likely allow a certain amount of current discharge from the steel without metal loss. The critical factor in this is the resupply of the alkalinity relative to the current drain.
Instances of stray current corrosion occurring have been recorded. An example was a jetty where the piles were being cathodically protected, and the reinforced concrete deck was being used as the system negative.
Unfortunately, several of the piles were electrically discontinuous, and corrosion occurred at a secondary anode point formed on these piles as the current attempted to flow back to the system negatively.
The vast majority of the potential gradients found between different areas of the steel in concrete are caused by the existence of physical differences or nonuniformities on the surface of the steel reinforcement (different steels, welds, active sites on the steel surface, oxygen availability, chloride contamination).
These potential gradients can allow significant electrical current to flow and cause, under certain circumstances, i.e., with aggressive ions in the concrete, corrosion of the reinforcement.
Even though the potential for electrochemical corrosion might exist because of the non-uniformity of the steel in the concrete.
This corrosion is usually prevented even at nominally (i.e., more harmful in potential than cathodic area) anodic sites by the passivated film, which is found on the steel surface in the presence of moisture, oxygen, and water-soluble alkaline products formed during the hydration of the cement.
There are two mechanisms by which the highly alkaline environment and accompanying passivation effect may be destroyed, namely:
- The reduction of alkalinity by the leaching of alkaline substances by water or partial neutralization when reacting with carbon dioxide or other acidic materials.
- Electrochemical action involves aggressive ions acting as catalysts (typically chloride) in the presence of oxygen.
Reduction of alkalinity by reaction with carbon dioxide, as present either in the air or dissolved in water, involves neutralizing reactions with the sodium and potassium hydroxides and, subsequently, the calcium system, which is part of the concrete matrix.
This process—called carbonation—although progressing increasingly slowly, may in time penetrate the concrete to a depth of 25mm or so (depending upon the quality of the concrete and other factors) and thereby neutralize the protective alkalinity normally afforded to steel reinforcement buried to a lesser depth than this.
This damage is particularly apparent in low-grade concrete structures where the builders were economical with the cement and liberal with the water.
The second mechanism where the passivity of the steel in the concrete can be disrupted is by electrochemical action involving chloride ions and oxygen.
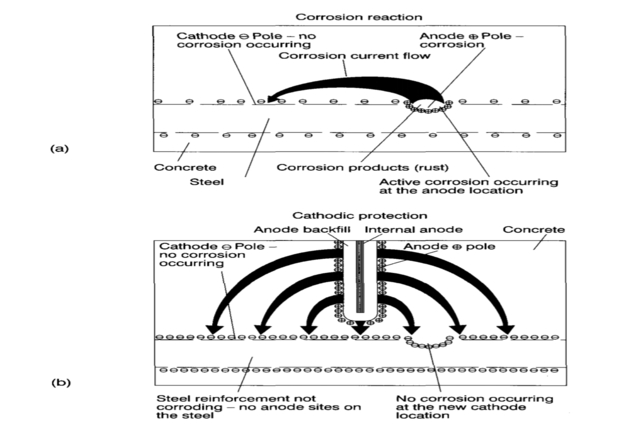
As previously mentioned, this is by far the most important degradation mechanism for reinforced concrete structures, and the most significant factors influencing this reaction are discussed below.
Also Read: The Contractor | Types, Responsibilities, and Conditions You Need To Know
Alkalinity and chloride concentrations:
The high alkalinity of the chemical environment normally present in concrete protects the embedded steel because of the formation of a protective film which could be either an oxide or a hydroxide, or even something in the middle, depending on which research paper you read.
This film’s integrity and protective quality depend upon the environment’s alkalinity (pH). The bulk alkalinity of the concrete depends on the water-soluble alkaline products.
The principal soluble product is calcium hydroxide, and the initial alkalinity of the concrete is at least that of saturated lime water (pH of about 12.4, depending upon the temperature).
In addition, there are relatively small amounts of sodium and potassium oxides in the cement which can further increase the alkalinity of the concrete or paste extracts, and pH values of 13.2 and higher have been reported.
The higher the alkalinity, the greater the protective quality of this film. Steel in concrete becomes potentially more susceptible to corrosion as the alkalinity is reduced.
Also, steel in concrete becomes more at risk with increasing quantities of soluble chlorides present at the iron-cement paste interface. Chloride ions appear to be a specific destroyer of the protective oxide film.
Chloride can be present in the ‘as-manufactured’ concrete as a set accelerator (calcium chloride) or through contamination of the mix, but more commonly, the chloride has come from an external source such as de-icing salts or marine environments.
In these latter cases, the salt diffuses through the concrete cover to the steel. It is worth noting that in practice, the rate of diffusion in an exposed marine or de-icing salt environment can be much higher than might be estimated when using cement or concrete disc ion diffusion tests. This is because the transport process utilizes convection and capillary movements in real structures.
Although chloride ions are soluble in the cement paste, most of the chlorides will not be in solution in the liquid within the paste. This is because the chlorides react with hydrated tricalcium sulfoaluminate, a constituent of the past, to produce a corresponding tricalcium chloroaluminate compound.
It has been shown that as much as 75–90% of the chloride in the cement paste exists in the chloroaluminate compound and is thus ‘bound’ and unable to interact with the steel reinforcement.
This concentration depends upon the total amount of chloride present, the tricalcium aluminate (C3A) content, and the degree of hydration of the cement.
Although only a fraction of the chloride is in solution, it is in a dynamic equilibrium. Thus, the chloroaluminate compound present would allow resupply if the free chloride was leached out.
The relationship between the onset of steel corrosion and the alkalinity and chloride concentration in the environment has not been fully defined for in-situ concrete.
It is unlikely to be so without a definition of the aggregate and cement types and quantities. It has been suggested and seems reasonable that a threshold concentration of chloride ions must be exceeded in an oxygen-rich environment before a significant level of steel reinforcement corrosion occurs. Some of the defined values for this level are given in the Table below.
Authority | % of Cl by weight of cement |
Wegler | 0.40 |
BS CP11 1979 | 0.36 |
Clear | 0.20 |
Knofel | 0.20 |
ACI Committee 201 | 0.20 – 0.10 |
Vassie | 0.10 |
As can be seen, there is a very large range of defined critical values. Part of the reason is that a scientist would probably define the onset of corrosion when sufficient chloride is at the rebar to catalyze the corrosion reaction.
In contrast, if there is a low corrosion rate in a site investigation of commercial structures, then visual inspection would infer that no corrosion was occurring.
This observation would allow the erroneous conclusion that the threshold had not been reached. Whatever the critical concentration level, it appears certain that both the structural environment and the concrete significantly affect the critical concentration of chlorides required to get corrosion properly underway.
A hydroxide and chloride concentrations ratio can more accurately describe these critical values. This has been given among others by Hausmann (1967) as:
It is sometimes found that reinforced concrete with uniformly high levels of chloride contamination (often over 3%) does not have significant corrosion of the rebar.
This typically happens when there are constant environmental conditions around the concrete, for example, internal walls of a building or structures buried below a saline water table.
Conversely, in areas where there are cyclical environmental conditions, such as where concrete is exposed to strong tidal flows of aerated salt water or where there are daily weather conditions, e.g., where there is direct sunlight in the day and high humidity and low temperatures in the night, there can be significant damage at low chloride concentrations.
Various ideas on what chloride is doing to cause this depassivation have been proposed, but there seems to be agreement that in localized areas, the passive film is broken down with pitting resulting.
In the pits, an acid environment exists, and when concrete is stripped from the corrosion sites on the steel, green-black and yellow-black compounds can often be observed.
These are probably intermediate complexes that contain chloride and allow lower activation energy for oxidation. In the corrosion process, chloride is not held as a final product and can be thought of as acting as a catalyst.
Any increase in chloride ion concentration beyond the initiation level will likely increase the corrosion rate. At some point, other factors will become the rate-limiting step. This rate-limiting step in reinforced concrete is commonly the availability of sufficient oxygen.
Oxygen level:
An essential factor for the corrosion of steel in concrete is the presence of oxygen at the steel-to-cement paste interface. Oxygen is required in addition to chloride or reduced alkalinity.
If oxygen is not present, then there should not be any oxidation. For example, seawater has been used successfully as mixing water for reinforced concrete, continually and completely submerged in seawater at this seabed.
This is because of the maintenance of high alkalinity due to the sodium chloride (this boosts the concrete’s alkalinity due to sodium ions’ higher solubility in the cement paste), low oxygen content in the sea-water at the seabed, and the very slow diffusion rate of oxygen through the water-saturated paste.
There would initially be a high corrosion rate when the critical chloride ratio was achieved. This would deplete the available oxygen, and the corrosion rate would dramatically reduce, despite an increasing chloride concentration.
This slowing of the corrosion rate is assisted by a reduction in the oxygen solubility of water at very high chloride saturation levels, which would further reduce oxygen availability.
In most cases, when the structure is submerged, the oxygen diffusion process is the rate-controlling step in the speed of the corrosion.
The level of oxygen supply or resupply also has an effect on the corrosion products formed. A black product (magnetite) is formed in low oxygen availability, and a red-brown material (haematite) is favored in high oxygen availability.
The pore sizes of these oxides are different, with the red product forming a more open structure with bigger pores. The formation of haematite imparts a higher bursting pressure on the concrete because of its greater volume.
It allows a quicker reaction because of its greater porosity relative to magnetite. For these reasons, the presence of haematite rather than magnetite tends to indicate general corrosion rather than pitting and vice versa.
Cement type:
The concrete composition significantly affects the corrosion damage at a chloride concentration. One example is that hardened concrete appears to have a lower chloride tolerance level than concrete contaminated during mixing.
This is practically evident in pre-cast units, which tend to corrode less than might be anticipated even when heavily dosed with a calcium chloride set accelerator (this is probably at least partially explainable due to their higher quality relative to cast in-situ reinforced concrete of the same vintage and the absence of any potential differences caused by concentration gradients).
Although cement composition and type can affect corrosion, this effect is relatively small compared to the concrete quality, cover over the steel, and concrete consolidation.
Using cement with a high C3A content will tend to bind more chlorides and thus reduce the amount of chloride, freeing to disrupt the oxide film on the steel reinforcement.
Also, a cement of high alkali content would appear to offer advantages because of the higher inherent alkalinity provided. In general, it is observed that cement high in C3A affords more excellent corrosion protection to reinforcing steel, but it is thought that other factors, such as fineness and sulfate content, may have at least as significant an effect.
One study by Tuutti (1982) found that Portland cement had a higher initiation level than slag cement, but a lower diffusion resistance and thus postulated that in certain exposure conditions, a certain mix design with Portland cement would be superior, while under other conditions, the reverse was true and a slag cement would be superior.
It was noted that sulfate-resisting cement was always less effective than Portland cement.
Aggregate type and other additives:
n general, the higher the strength of the aggregate, the more likely it is to be resistant to the passage of ions. But this is not always so.
For example, granite aggregate has been used for several significant projects because of its high strength, but concrete made with this material has been found to provide relatively poor diffusion resistance results. This is probably because of microcracks in the aggregate.
Likely, a substantial amount of the diffusion in concrete proceeds along the interface between the aggregate and the cement paste. This region may prove to be more critical than the bulk diffusion resistance of the aggregate. Certain aggregates have a smoother profile than others, affecting the apparent diffusion path.
Adding additives, such as microsilica, to the concrete is beneficial as it increases the diffusion path by tending to block the pores in the concrete.
The only real problem with this and other additives is the additional care required when cast on the construction site and the assumption that the concrete will change into an impermeable covering with additive X. This is not a safe assumption, as the concrete will still retain a degree of porosity.
We hope this article helped you learn about Corrosion in Reinforced Concrete: Causes, Prevention, and More. You may also want to learn about Construction Estimating: Types, Opportunities, The Estimator, and More, 2022 Top 10: Our Most Popular Posts of the Year, Engineering Universities In Tanzania You Should Know, and Cement | Types, Composition, And All You Need to Know.
If you liked this article, please Join WebsiteForEngineers on Telegram, and you can also find us on Pinterest, Twitter, and Facebook.